Where BrainEye could help
improve health outcomes
Brain health
Head trauma
Cognitive decline
Drug and alcohol
impairment
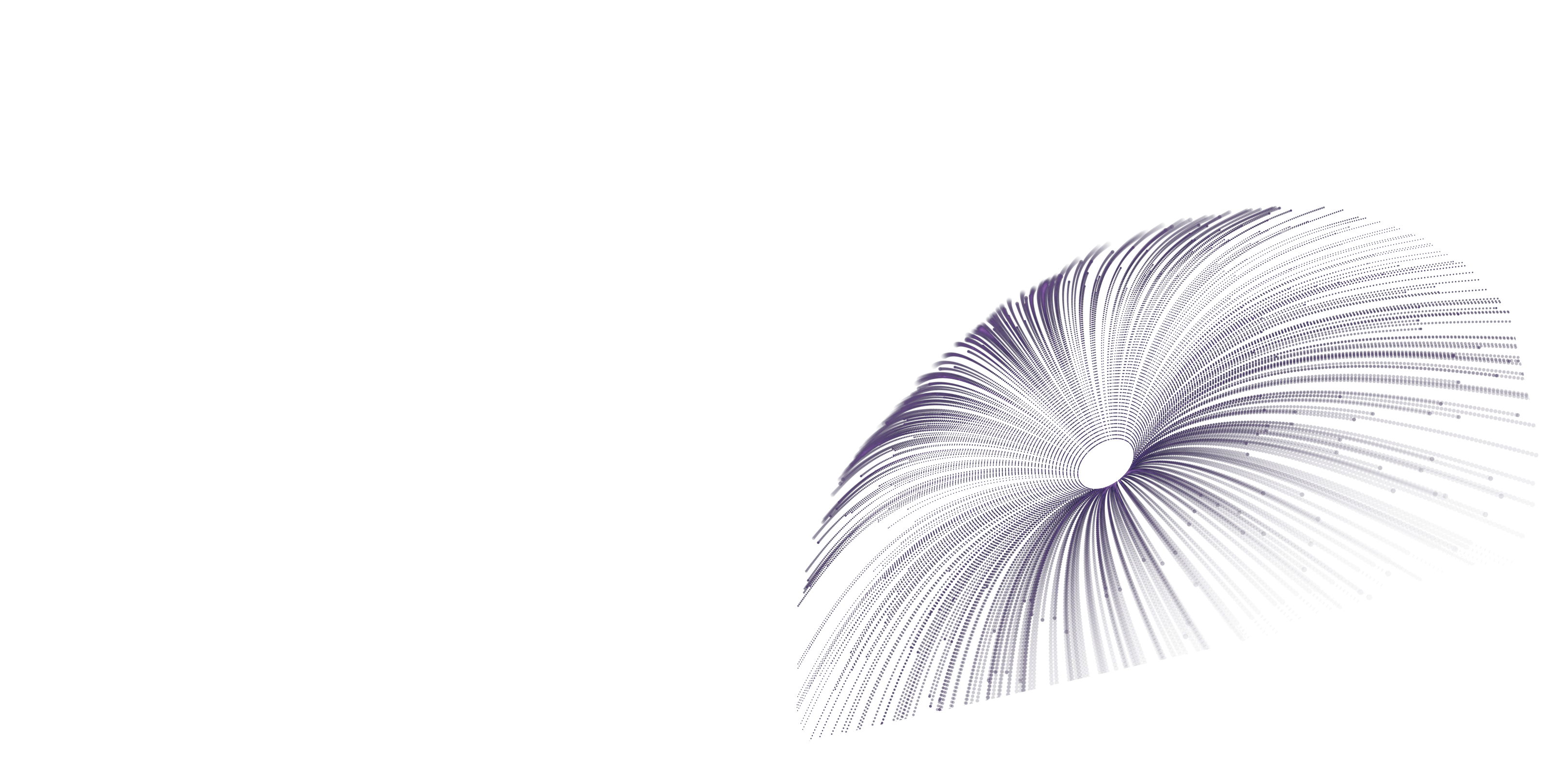
Why the Eye?
The problem
- Conventional neurological examination is limited and subjective,
evaluating ~10% of brain - Neuropsychological examination is costly/not readily accessible
The solution
- Assessment of the visual-ocular motor system
- Evaluates >50% of the brain (direct connections)
- Affordable, accessible
- Damage at any point = measurable abnormalities in eye movement
Current in-app tests
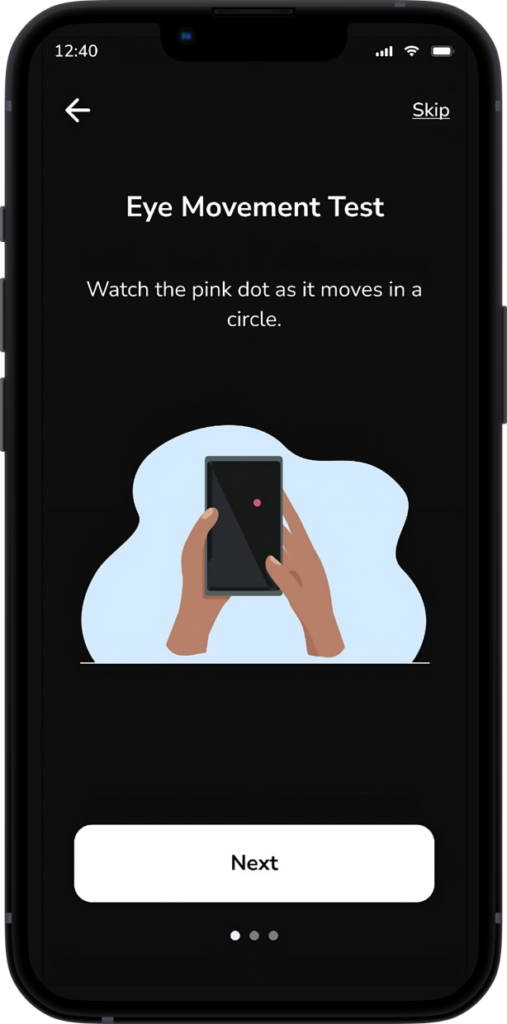
Smooth Pursuit (SMP)
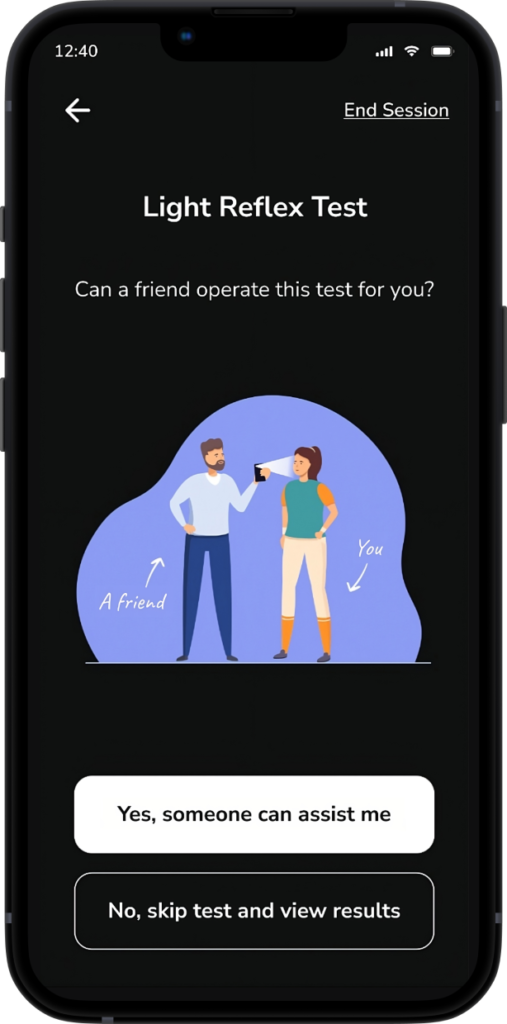
Pupillary Light Reflex (PLR)
Application within a broader
brain health context
Application within a broader brain health context
Given the breadth of the circuitry involved in the control of vision and eye movements it is perhaps not surprising that SMP and PLR changes are seen in many neurological conditions.
Smooth Pursuit (SMP)
Although non-specific, SMP eye movement abnormalities are evident in a large number of patient populations/neuropathologies consistent with the extent of the system supporting their integrity. Using different types of stimuli (circular horizontal, vertical, sinusoidal, step ramp), velocities and stimuli sizes/shapes, SMP changes include:
- Multiple sclerosis – reduced gain, increased latencies, high amplitude saccades [1]
- Parkinson’s disease – reduced gain, inadequate catch up saccades [2]
- Multiple System Atrophy – reduced gain, catch up and/or anticipatory saccades [3]
- Progressive Supranuclear Palsy – reduced range vertically, many catch up saccades vertically [3]
- Alzheimer’s disease – reduced peak velocities, increased number and amplitude of anticipatory saccades [4]
- Cerebellar ataxias (not SCA7 or 2) – saccadic pursuit [5]
- Amyotrophic Lateral Sclerosis – square wave jerk, saccadic pursuit [6]
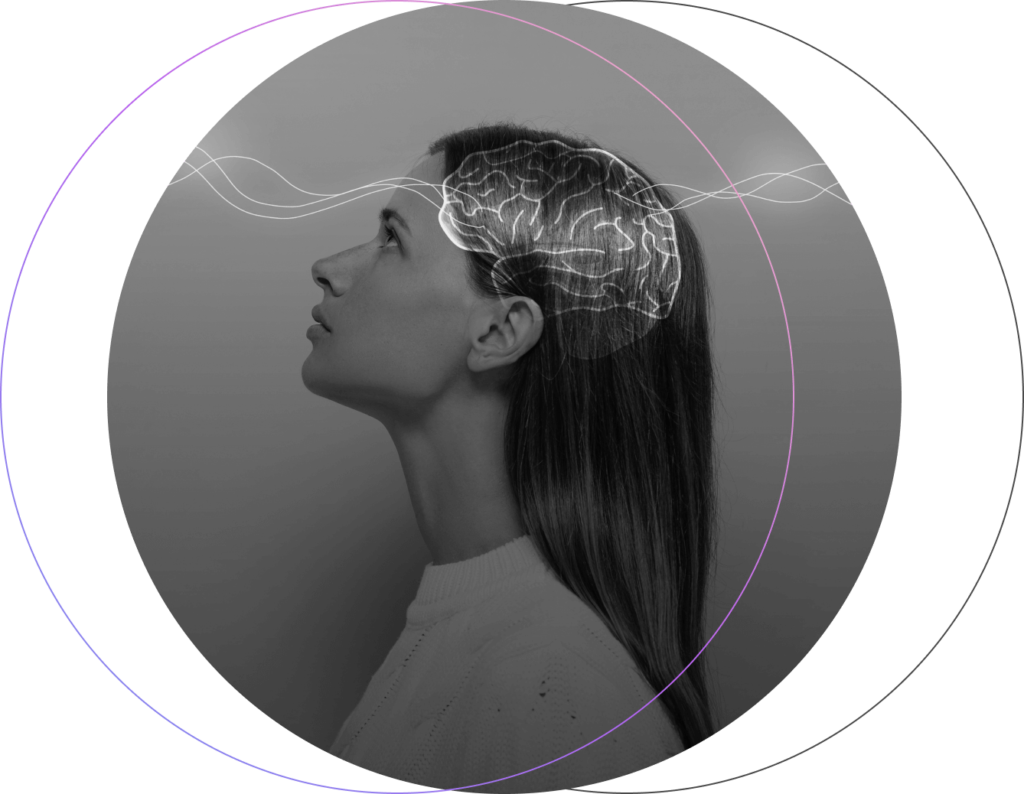
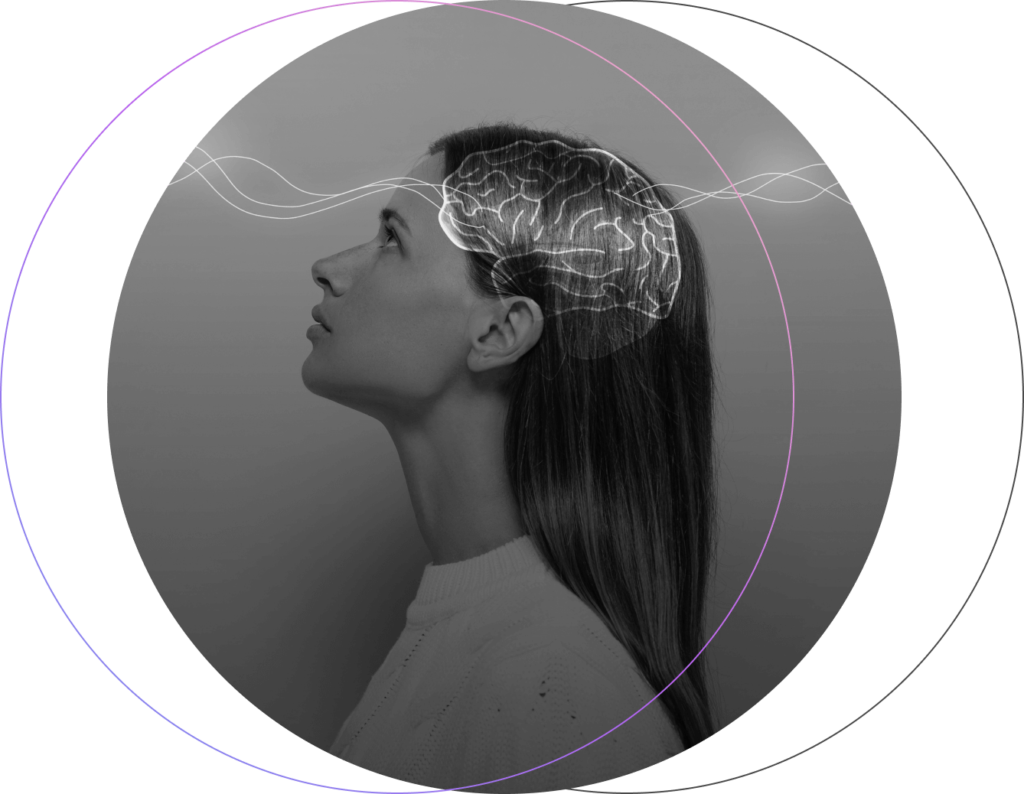
Smooth Pursuit (SMP)
SMP eye movements implicate a complex neural system with long ranging brain connections spanning the visual pathways from retina to cortex, brainstem, cerebellum, basal ganglia and multiple visual, temporal, parietal and frontal cortical regions. Given the complexity of the system, it is perhaps unsurprising that a range of SMP metrics distinguish concussed from non-concussed individuals. Although studies have used several types of stimuli (circular horizontal, vertical, sinusoidal, step ramp), many different velocities and stimuli sizes/shapes, results variously demonstrate that following a concussion, SMP eye movements may:
- be inaccurate (e.g. lag behind a moving target with reduced velocity) [50-56]
- be dysconjugate (eyes movements are asymmetrical) [52, 57, 58]
- have increased initiation latencies [53]
- be interrupted by saccades (or feature many catch up saccades) [54, 55]
- show greater intra-individual variability in accuracy [56]
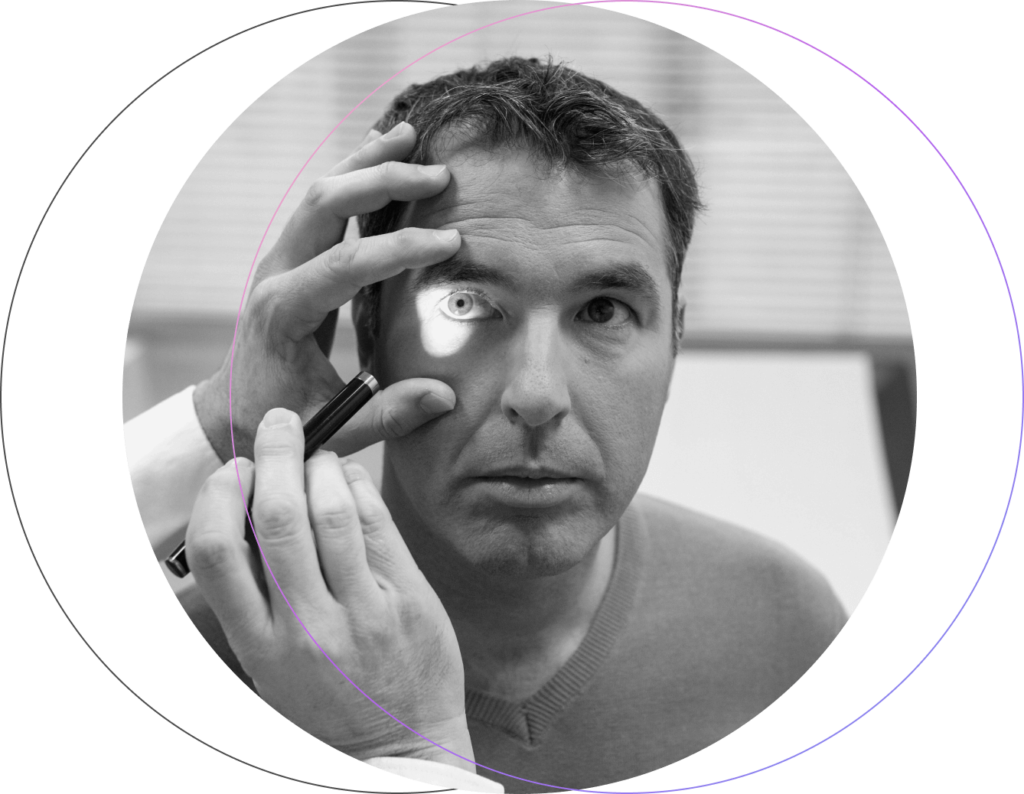
Pupillary Light Reflex (PLR)
PLR changes may be found in a myriad of contexts including:
- Optic nerve injury
- Oculomotor nerve damage
- Brain stem lesions, such as tumours
- Concussion
- Medications that dilate the pupil (mydriasis) → sympathomimetics (e.g. phenylephrine, adrenaline) and antimuscarinics (e.g. cyclopentolate, tropicamide, atropine), tricyclic antidepressants, amphetamines and ecstasy [7]
- Medications that constrict the pupil (miosis) → muscarinic agonists (e.g. pilocarpine) and opiates (e.g. morphine) [7]
- PLR changes have also been recently taken into consideration as potential markers of Alzheimer’s disease → decreased maximum velocity of constriction and maximum constriction acceleration [8]
Published evidence
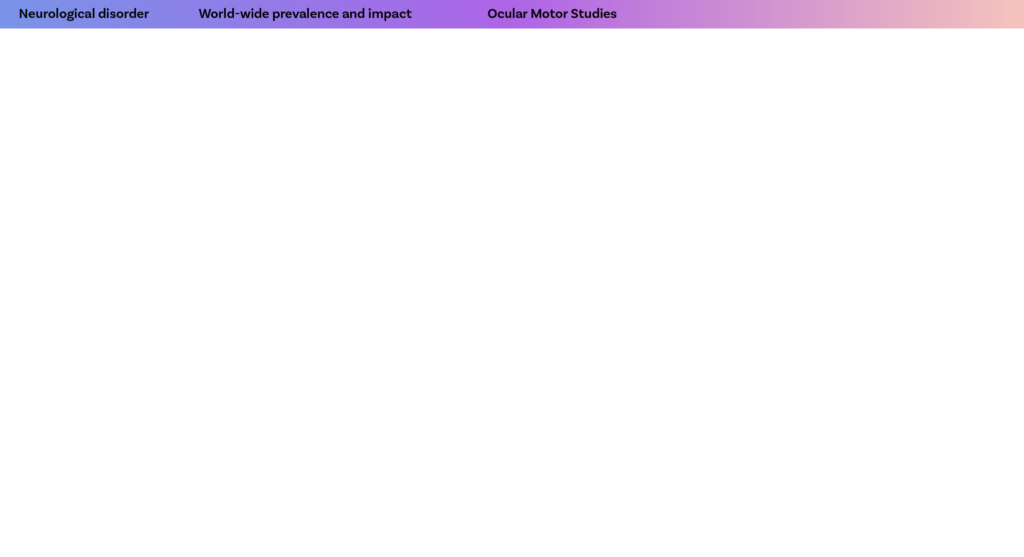
*A/Prof Joanne Fielding (Chief Scientific Officer, BrainEye) & Prof Owen White (Chief Medical Officer, BrainEye)
Sports industry spotlight
Why better concussion diagnosis and management is critical
Around 50% of concussions go unreported or undiagnosed [44]
Brain swelling from second hit syndrome has mortality rates close to 50% and morbidity rates at 100% [45]
The utility of eye movements in
measuring concussion
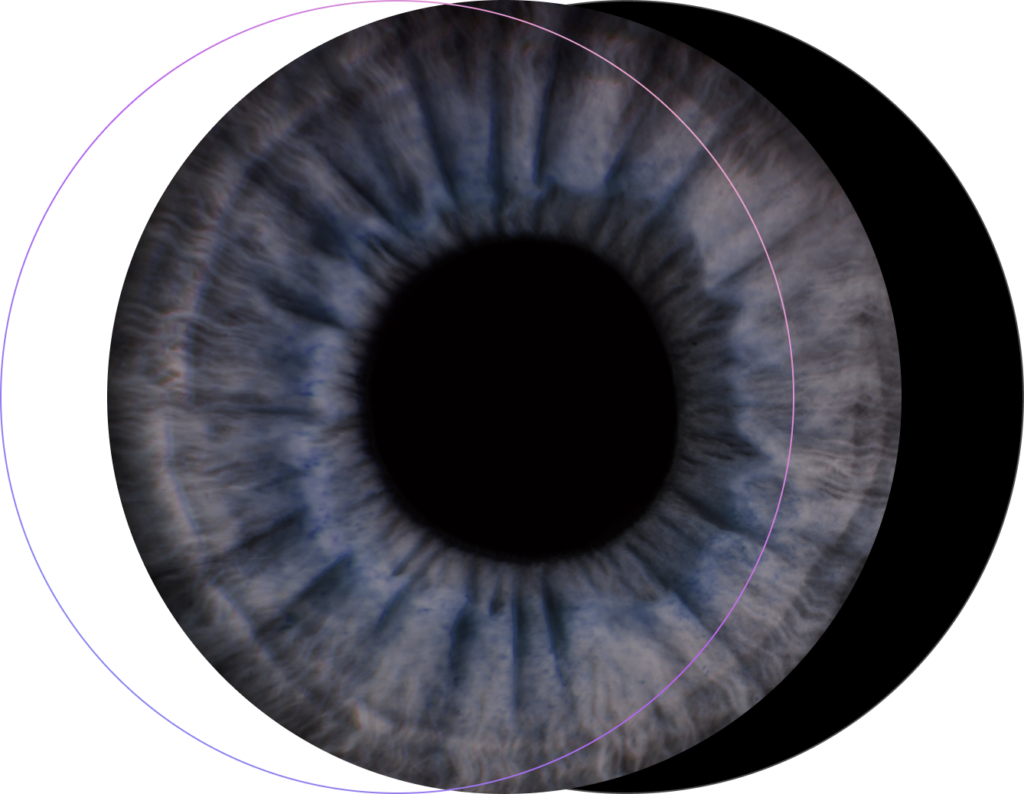
Pupillary Light Reflex (PLR)
Pupil abnormalities are common immediately following a concussion and while they often resolve over a short period of time, can persist indefinitely. Notably, pupillary size, shape, and reactivity to light are regulated by the autonomic nervous system – central autonomic control nuclei and pathways are mainly integrated within the brainstem, shown in histopathological studies to be an important site of axonal injury following concussion. Although studies have tested subjects with different stimulus conditions, at various ages, at various time points following concussion, results variously demonstrate that following a concussion, pupillary changes to:
- Steady-state pupil size [46-48]
- Pupil size following constriction in response to a light stimulus [46-48]
- Latencies (time to maximum constriction in response to a light stimulus) [48]
- Peak and average constriction velocities [46-49]
- Peak and average dilation velocities [46, 48, 49]
- T75 (time to 75% pupillary redilation) [49]
- Constriction amplitudes [46]
Smooth Pursuit (SMP)
SMP eye movements implicate a complex neural system with long ranging brain connections spanning the visual pathways from retina to cortex, brainstem, cerebellum, basal ganglia and multiple visual, temporal, parietal and frontal cortical regions. Given the complexity of the system, it is perhaps unsurprising that a range of SMP metrics distinguish concussed from non-concussed individuals. Although studies have used several types of stimuli (circular horizontal, vertical, sinusoidal, step ramp), many different velocities and stimuli sizes/shapes, results variously demonstrate that following a concussion, SMP eye movements may:
- be inaccurate (e.g. lag behind a moving target with reduced velocity) [50-56]
- be dysconjugate (eyes movements are asymmetrical) [52, 57, 58]
- have increased initiation latencies [53]
- be interrupted by saccades (or feature many catch up saccades) [54, 55]
- show greater intra-individual variability in accuracy [56]
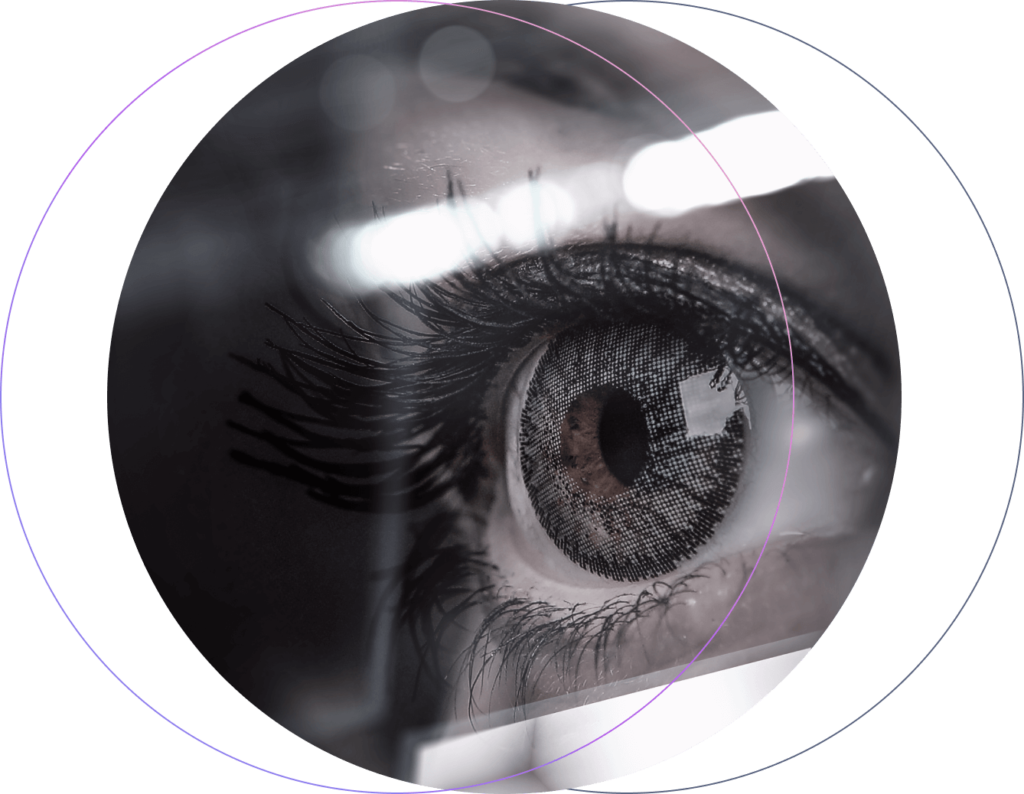
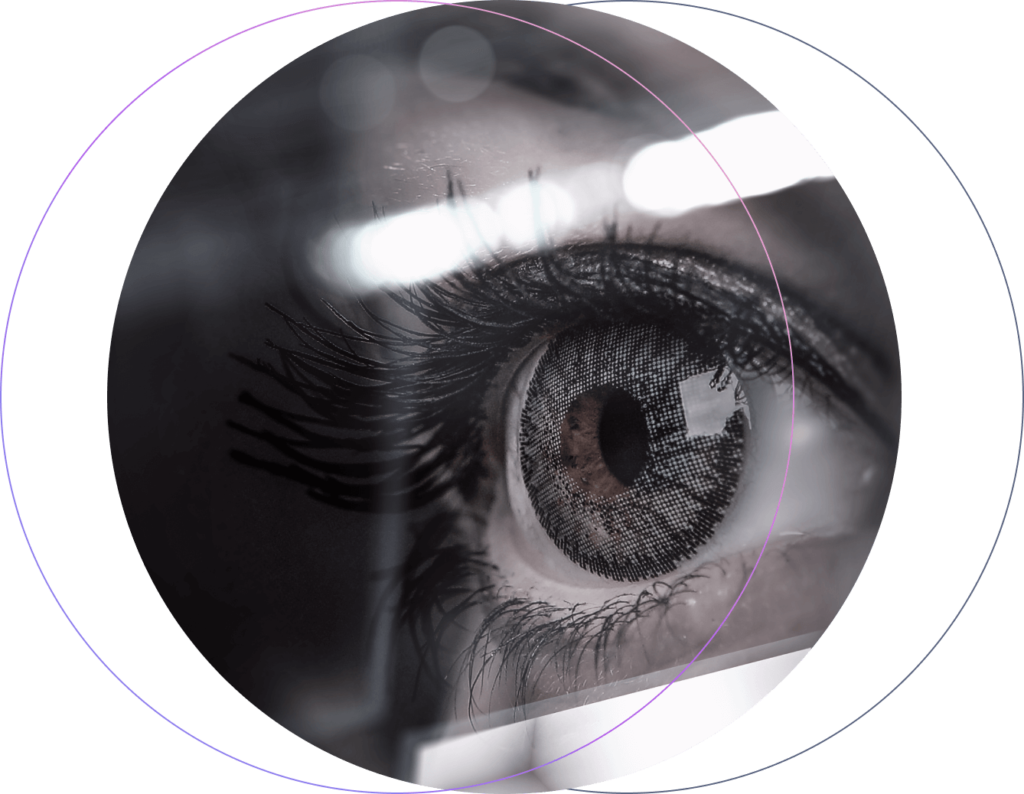
Smooth Pursuit Eye movements (SMP)
Although non-specific, SMP eye movement abnormalities are evident in a large number of patient populations/neuropathologies consistent with the extent of the system supporting their integrity. Using different types of stimuli (circular horizontal, vertical, sinusoidal, step ramp), velocities and stimuli sizes/shapes, SMP changes include:
- Multiple sclerosis – reduced gain, increased latencies, high amplitude saccades [1]
- Parkinson’s disease – reduced gain, inadequate catch up saccades [2]
- Multiple System Atrophy – reduced gain, catch up and/or anticipatory saccades [3]
- Progressive Supranuclear Palsy – reduced range vertically, many catch up saccades vertically [3]
- Alzheimer’s disease – reduced peak velocities, increased number and amplitude of anticipatory saccades [4]
- Cerebellar ataxias (not SCA7 or 2) – saccadic pursuit [5]
- Amyotrophic Lateral Sclerosis – square wave jerk, saccadic pursuit [6]
Want to know more?
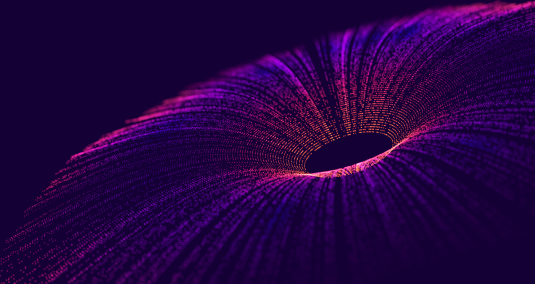
References
- Lizak, N., et al., Impairment of Smooth Pursuit as a Marker of Early Multiple Sclerosis. Front Neurol, 2016. 7: p. 206.
- Frei, K., Abnormalities of smooth pursuit in Parkinson’s disease: A systematic review. Clin Park Relat Disord, 2021. 4: p. 100085.
- Pinkhardt, E.H., et al., Comparison of smooth pursuit eye movement deficits in multiple system atrophy and Parkinson’s disease. J Neurol, 2009. 256(9): p. 1438-46.
- Zaccara, G., et al., Smooth-pursuit eye movements: alterations in Alzheimer’s disease. J Neurol Sci, 1992. 112(1-2): p. 81-9.
- Stephen, C.D. and J.D. Schmahmann, Eye Movement Abnormalities Are Ubiquitous in the Spinocerebellar Ataxias. Cerebellum, 2019. 18(6): p. 1130-1136.
- Guo, X., et al., Eye Movement Abnormalities in Amyotrophic Lateral Sclerosis. Brain Sci, 2022. 12(4).
- Belliveau, A.P., A.N. Somani, and R.H. Dossani, Pupillary Light Reflex, in StatPearls. 2022: Treasure Island (FL).
- Chougule, P.S., et al., Light-Induced Pupillary Responses in Alzheimer’s Disease. Frontiers in Neurology, 2019. 10.
- Global, regional, and national burden of multiple sclerosis 1990-2016: a systematic analysis for the Global Burden of Disease Study 2016. Lancet Neurol, 2019. 18(3): p. 269-285
- Global, regional, and national burden of Parkinson’s disease, 1990-2016: a systematic analysis for the Global Burden of Disease Study 2016. Lancet Neurol, 2018. 17(11): p. 939-953
- Global, regional, and national burden of Alzheimer’s disease and other dementias, 1990-2016: a systematic analysis for the Global Burden of Disease Study 2016. Lancet Neurol, 2019. 18(1): p. 88-106.
- Charlson, F.J., et al., Global Epidemiology and Burden of Schizophrenia: Findings From the Global Burden of Disease Study 2016. Schizophr Bull, 2018. 44(6): p. 1195-1203.
- Spinal Cord Injury, Global, regional, and national burden of traumatic brain injury and spinal cord injury, 1990-2016: a systematic analysis for the Global Burden of Disease Study 2016. Lancet Neurol, 2019. 18(1): p. 56-87
- Global, regional, and national burden of stroke, 1990-2016: a systematic analysis for the Global Burden of Disease Study 2016. Lancet Neurol, 2019. 18(5): p. 439-458.
- Global, regional, and national burden of migraine and tension-type headache, 1990-2016: a systematic analysis for the Global Burden of Disease Study 2016. Lancet Neurol, 2018. 17(11): p. 954-976.
- Kondziella, D., M.H. Olsen, and J.P. Dreier, Prevalence of visual snow syndrome in the UK. Eur J Neurol, 2020. 27(5): p. 764-772
- Clough, M., et al., Cognitive processing speed deficits in multiple sclerosis: Dissociating sensorial and motor processing changes from cognitive processing speed. Mult Scler Relat Disord, 2019. 38: p. 101522.
- Clough, M., et al., Multiple sclerosis: Executive dysfunction, task switching and the role of attention. Mult Scler J Exp Transl Clin, 2018. 4(2): p. 2055217318771781.
- Clough, M., et al., Ocular Motor Measures of Cognitive Dysfunction in Multiple Sclerosis I: Inhibitory Control. Journal of Neurology, 2015. 262(5): p. 1130-1137.
- Clough, M., et al., Ocular Motor Measures of Cognitive Dysfunction in Multiple Sclerosis II: Working Memory. Journal of Neurology, 2015. 262(5): p. 1138-1147.
- Fielding, J., et al., Ocular motor signatures of cognitive dysfunction in multiple sclerosis. Nat Rev Neurol, 2015. 11(11): p. 637-45.
- Fielding, J., et al., Longitudinal assessment of antisaccades in patients with multiple sclerosis. PLoS One, 2012. 7(2): p. e30475.
- Fielding, J., et al., Control of visually-guided saccades in Multiple Sclerosis: Disruption to higher order processes. Neuropsychologia, 2009. 47: p. 1647–1653.
- Fielding, J., et al., Antisaccade performance in patients with multiple sclerosis. Cortex, 2009. 45(7): p. 900-3.
- Fielding, J., et al., Multiple Sclerosis: cognition and saccadic eye movements. Journal of the Neurological Sciences, 2009. 277: p. 32-36.
- Kolbe, S.C., et al., Inhibitory saccadic dysfunction is associated with cerebellar injury in multiple sclerosis. Hum Brain Mapp, 2014. 35(5):2310-9.
- Ternes, A.M., et al., Characterization of inhibitory failure in Multiple Sclerosis: Evidence of impaired conflict resolution. J Clin Exp Neuropsychol, 2019. 41(3): p. 320-329.
- Fielding, J., et al., No sequence dependent modulation of the Simon effect in Parkinson’s disease. Brain Res Cogn Brain Res, 2005. 25(1): p. 251-60.
- Henderson, T., et al., Inhibitory control during smooth pursuit in Parkinson’s disease and Huntington’s disease. Mov Disord, 2011. 26(10): p. 1893-9.
- Fielding, J., et al., Temporal variation in the control of goal-directed visuospatial attention in basal ganglia disorders. Neurosci Res, 2006. 54(1): p. 57-65.
- Fielding, J., N. Georgiou-Karistianis, and O. White, The role of the basal ganglia in the control of automatic visuospatial attention. J Int Neuropsychol Soc, 2006. 12(5): p. 657-67.
- White, O.B., et al., Ocular motor deficits in Parkinson’s disease: I. The horizontal vestibulo-ocular reflex and its regulation. Brain, 1983. 106: p. 550-570.
- White, O.B., et al., Ocular motor deficits in Parkinson’s disease. II. Control of the saccadic and smooth pursuit systems. Brain, 1983. 106: p. 571-587.
- White, O.B., et al., Ocular motor deficits in Parkinson’s disease. III. Coordination of eye and head movements. Brain, 1988. 111: p. 115-129.
- Winograd-Gurvich, C., et al., Self-paced saccades and saccades to oddball targets in Parkinson’s disease. Brain Res, 2006. 1106(1): p. 134-41.
- Winograd-Gurvich, C., et al., Negative symptoms: A review of schizophrenia, melancholic depression and Parkinson’s disease. Brain Research Bulletin, 2006. 70: p. 312-321.
- Winograd-Gurvich, C., et al., Inhibitory control and spatial working memory: a saccadic eye movement study of negative symptoms in schizophrenia. Psychiatry Res, 2008. 157(1-3): p. 9-19
- Clough, M., et al., Oculomotor Cognitive Control Abnormalities in Australian Rules Football Players with a History of Concussion. J Neurotrauma, 2018. 35(5): p. 730-738.
- Dong, W., et al., Ischaemic stroke: the ocular motor system as a sensitive marker for motor and cognitive recovery. J Neurol Neurosurg Psychiatry, 2013. 84(3): p. 337-41.
- Solly, E., et al., Eye movement characteristics are not influenced by psychiatric comorbidities in people with visual snow syndrome. Brain Research, 2023. 1804:148265
- Foletta, P., et al., Delayed onset of Inhibition of Return in Visual Snow Syndrome. Frontiers in Neurology, 2021. 12:738599
- Solly, E., et al., Eye movement characteristics provide an objective measure of visual processing changes in patients with Visual Snow Syndrome. Scientific Reports, 2021. 11(1):9607
- Solly, E., et al., Ocular motor measures of visual processing changes in visual snow syndrome. Neurology, 2023. 95(13)
- Asken., B., et al., Playing through it”: Delayed reporting and removal from athletic activity after concussion predicts prolonged recovery. Journal of Athletic Training, 2016. 51(4): 329–335.
- Martineau, C., et al., Guidelines for treatment of sport-related concussions. Journal of the American Academy of Physician Assistants, 2007. 20 (5), p.22-27
- Thiagarajan, P. and K.J. Ciuffreda, Pupillary responses to light in chronic non-blast-induced mTBI. Brain Inj, 2015. 29(12): p. 1420-5.
- Master, C.L., et al., Utility of Pupillary Light Reflex Metrics as a Physiologic Biomarker for Adolescent Sport-Related Concussion. Jama Ophthalmology, 2020. 138(11): p. 1135-1141.
- Truong, J.Q. and K.J. Ciuffreda, Comparison of pupillary dynamics to light in the mild traumatic brain injury (mTBI) and normal populations. Brain Injury, 2016. 30(11): p. 1378-1389.
- Capo-Aponte, J.E., et al., Validation of Visual Objective Biomarkers for Acute Concussion. Mil Med, 2018. 183(suppl_1): p. 9-17.
- Murray, N.G., et al., Smooth Pursuit and Saccades after Sport-Related Concussion. J Neurotrauma, 2020. 37(2): p. 340-346.
- DiCesare, C.A., et al., Quantification and analysis of saccadic and smooth pursuit eye movements and fixations to detect oculomotor deficits. Behav Res Methods, 2017. 49(1): p. 258-266.
- Maruta, J., et al., Visual Tracking Synchronization as a Metric for Concussion Screening. Journal of Head Trauma Rehabilitation, 2010. 25(4): p. 293-305.
- Kelly, K.M., et al., Oculomotor, Vestibular, and Reaction Time Effects of Sports-Related Concussion: Video-Oculography in Assessing Sports-Related Concussion. The Journal of Head Trauma Rehabilitation, 2019. 34(3): p. 176-188.
- Hunfalvay, M., et al., Smooth Pursuit Eye Movements as a Biomarker for Mild Concussion within 7-Days of Injury. Brain Injury, 2021. 35(14): p. 1682-1689.
- Danna-Dos-Santos, A., et al., Long-term effects of mild traumatic brain injuries to oculomotor tracking performances and reaction times to simple environmental stimuli. Sci Rep, 2018. 8(1): p. 4583.
- Suh, M., et al., Deficits in predictive smooth pursuit after mild traumatic brain injury. Neurosci Lett, 2006. 401(1-2): p. 108-13.
- Hoffer, M.E., et al., The use of oculomotor, vestibular, and reaction time tests to assess mild traumatic brain injury (mTBI) over time. Laryngoscope Investig Otolaryngol, 2017. 2(4): p. 157-165.
- Samadani, U., et al., Eye tracking detects disconjugate eye movements associated with structural traumatic brain injury and concussion. J Neurotrauma, 2015. 32(8): p. 548-56.